- A Business Case For Biodiversity -

Topics in this primer:
Why It Matters: Increasing genetic erosion of the global Cannabis Hemp gene pool
What is a landrace aka local ecotype?
Landrace / local ecotype populations have higher genetic diversity
Key differences of landraces vs. cultivars: yield stability & resilience adaptation
Landraces adapt better to environmental stresses & pressures
Differences in traits between wild-type vs. domesticated cannabis
Conserving, protecting & regenerating landrace genetic diversity
Introduction
Here at Motor City Seeds, part of our mission is to support and promote biodiversity & resilience, landraces aka local ecotypes, and the conservation and regeneration of germplasm & genetic diversity (including the origin habitats/ecosystems where genetic diversity germplasm material is sourced/obtained from).
We’ve prepared this primer to help more people understand and appreciate the benefits and importance of landraces aka local ecotypes (i.e., native, indigenous, and/or or locally-adapted/naturalized species in either wild-growing populations and/or varieties maintained over generations by local farmers in concert with the natural selective pressures of the local environment) and how the choices you make (when shopping for cannabis seeds and while growing your plants) can help contribute to the global movement to preserve, protect and regenerate our planet’s cannabis hemp genetic diversity & global gene pool.

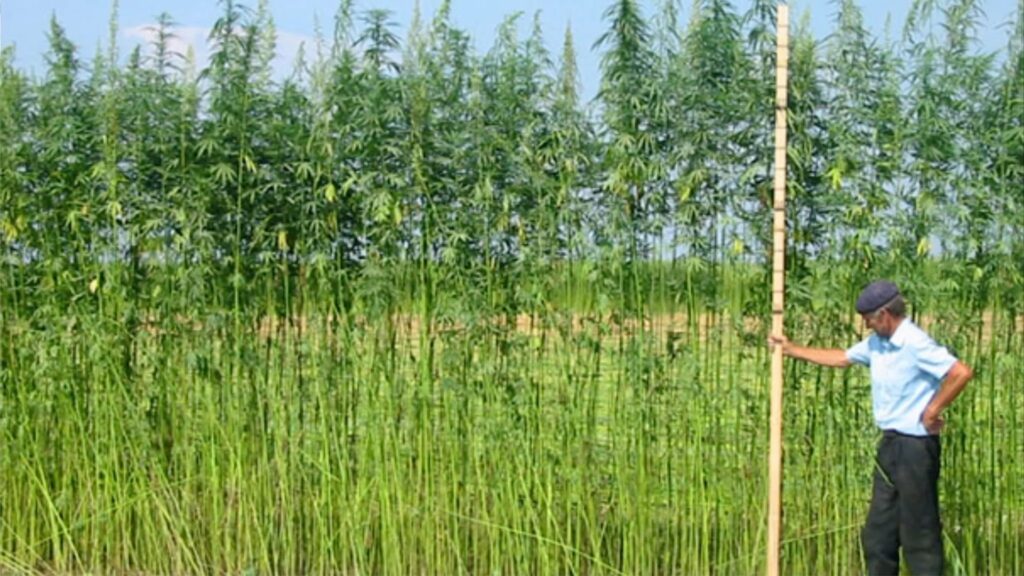
Why It Matters: Increasing genetic erosion of the global Cannabis Hemp gene pool
Genetic erosion (via genetic bottlenecking) has significantly reduced the total genetic diversity of the global cannabis hemp population / gene pool, which reduces resilience and increases the risk of future harvests being impacted by climate change and increased intensity/variation/unpredictability of other environmental pressures (water availability, salinity/soil erosion, changes in rainfall temperature patterns & growing-season length, industrial toxins etc.):
The growing increase of human population and environmental stresses have led to land use changes and habitat destruction (of habitat formerly occupied by landrace and/or wild cannabis) in order to produce a greater amount of food.


The advent of high input and intensive monoculture systems (including within the booming cannabis/hemp industry), based on genetically similar individuals (elite cultivars, pure lines, hybrids, or clones) improved for particular traits (i.e., high yield and better end-use quality) and derived from an increasingly narrow germplasm pool has resulted in a loss of potentially useful traits, reducing the genetic variability available to plant breeders for crop improvement.
To cope with climate change and ecosystem change and meet the needs of new varieties for marginal areas and new environments (for example, new homegrower outdoor gardens), researchers and breeders are constantly looking for new sources of genetic variability, a key source of which is represented by landraces.
Collection and conservation of germplasm of indigenous populations of cannabis and/or hemp landraces in their centers of diversity is urgently needed. The germplasm base outside landrace traditional centers of diversity has become genetically contaminated by both widespread crossbreeding and incestuous inbreeding. In the context of climate change, increasing unpredictability of environmental stressors, and unpredictable future needs, in situ conservation of agrobiodiversity (in native wild origin habitats and also on-farm/in-garden within the context of traditional low-input farming systems) is much preferable for crop plants and their wild relatives, but given the precarious continued existence of unaltered aboriginal wild populations of Cannabis, preservation in seed banks is also an immediate priority.
With regards to the global cannabis gene pool, we (the industry/global cannabis community as a whole) have increasingly become very narrow in what we’ve selected. We’ve wanted plants that had a lot of aroma, a lot of potency, matured early, and gave high yields. A lot of the landraces really didn’t give us that. That’s why we’ve gone on and adopted these hybrid varieties as being our standard these days. But, despite all the variety we see between let’s say a Kush vs. a Cookie or whatever, this is really looking at variety amongst a very tiny part of the whole cannabis gene pool: these incestuous super-hybrid strains are leaving out all the hemp varieties, a lot of the wild varieties or escaped varieties that are there, unselected types. And it’s getting harder and harder to go back and find the original building blocks any more. So we’re kind of stuck aka bottlenecked: the genes are there but they’ve assorted into what’s become popular today. And it’s hard to go back and find let’s say an original Colombian to get gene combinations from that plant that we’ve suppressed, that we’ve selected against for the last 30 years.
Genetic erosion is the main threat to landraces. What is genetic erosion? Genetic erosion has been referred to in the literature as:
the loss of a crop, variety or allele (gene/trait) diversity
the reduction in richness (in the total number of crops, varieties or alleles/traits)
the reduction in evenness (i.e. of genetic diversity)

When describing the amount of genetic diversity present in a crop, the three most important features measured are the richness, evenness and distinctness of the characteristics: Richness is a measure of the number of different types, while evenness describes their distribution within and between the different populations (cultivars, crop fields and/or home gardens, areas etc.). Distinctness provides useful additional information on how different the types are and can help assess whether some populations or areas have unique ecotypes.
Modern varieties are bred to be genetically uniform (they are often pure lines or F1 hybrids) to maximize production ability under those inputs that make the cultivation environment best suited to the crop (irrigation, fertilization, pest control, etc.) as well as to meet the increasing demands of mechanized harvesting and handling, and meet modern regulated industry/dispensary quality controls.

It is also because of their good yielding performances (at least in some agro-ecosystems) that modern varieties have taken over from the genetically variable, often lower yielding, locally adapted strains or landraces in the fields of farmers. Thus uniformity replaced diversity, and is still replacing it.
This alarms both geneticists and breeders, since lack of diversity severely impairs the future improvement of crops and/or limits the possibilities for facing new production constraints. Breeding for uniformity is an Achilles’ heel for the cultivars…
Selection activities during ongoing breeding programs led to a reduction of the diversity in genetic materials and are considered a bottleneck in crop evolution after the domestication process. This assumption is accompanied by the idea that many alleles (gene combinations that code for a particular trait) useful for breeding may have been lost in the selection process. The loss of genetic diversity within crop plants is believed to be extensive and therefore there has been increasing realization of the need to actively conserve the crop genepool, for all crop species in general and in particular cannabis hemp:
Many times throughout history, as humans migrated to new locales they most likely carried only a few seeds to a new settlement, and often only a limited number of individual plants (sometimes only one female exhibiting specific favorable traits) were selected as parents. This produced genetic bottlenecks that gave rise to populations with restricted genomes in new areas of Cannabis growth. When limited numbers of seeds were dispersed into isolated regions where Cannabis was not already growing, and the resulting small populations were brought under new selective pressures, strong founder effects influenced the evolution of these new Cannabis crops and in some cases their feral escapes. Only a few plants of limited genetic diversity established new populations which eventually evolved into local landraces.

As a result of millennia of dissemination and contact with differing human cultures worldwide, Cannabis plants have evolved huge genetic diversity (in both geographically isolated wild populations and traditional landraces) which is expressed in a remarkably wide range of phenotypes. When indigenous traditional farmers maintain localized landraces, they are preserving genetic diversity because landrace populations reproduce sexually, and thus allow for genetic recombination, mutation, and evolution under a combination of human & natural selection, presenting a continually-regenerating pool of novel phenotypes for natural and human selection.
However, narrow selection and strong founder effects have seriously attenuated i.e. sharply reduced genetic diversity: the numbers and ranges of traditional agricultural societies are diminishing worldwide, and many landraces are already extinct, which does not bode well for the future of the Cannabis gene pool. The loss of any genetic diversity threatens unique genes and allelic combinations, as well as lowering future evolutionary fitness for the genus as a whole and limiting the diversity of desirable products that crop plants may be able to provide.
Breeders’ well-intentioned domestication efforts have been strongly affected by the repercussions of prohibition and consequently its genetic diversity is increasingly dwindling, bringing us closer to potential genetic-monoculture disaster. A final blow to diversity may come from continued commercialization of a small number of select varieties that satisfy limited consumer preferences:
As a result of these trends, the status of the worldwide Cannabis genome has changed dramatically since the middle of the twentieth century: The domesticated Narrow-Leaf Drug hybrid cannabis varieties of the early and mid-1970s originated largely from crosses between Mexican or Jamaican landraces and more potent, but later ripening, Colombian, Indian, Panamanian, and/or Thai landraces. The rampant success of the increasingly-indoors/artificial-light cannabis production model narrowed the selection criteria of breeders toward developing only cultivars that flourished in relatively stable climate-controlled indoor grows, further limiting the present-day genetic diversity of drug Cannabis:

The majority of traditional fiber, seed, and drug landraces are no longer grown, and many of the few remaining in situ landraces have been genetically diluted through inter-breeding with introduced modern cultivars, and overall the genetic diversity of Narrow Leaf Hemp is currently very limited:
The vast majority of European industrial hemp cultivars are based on the same few single plant selections (Fibrimon pollen crossed with Russian & Chinese landraces); and the drug Cannabis gene pool has reached a genetic bottleneck caused by incest breeding between close relatives, intentional and unintentional selfing, highly directed selection for drug potency, and the preponderance of vegetatively reproduced (cloned) commercial populations. The genetic diversity of Cannabis is constantly reduced by persistent law enforcement seizures, accidental agricultural mishaps, and the gradual culling of economically unfavorable clonal lines: commercially valuable clones are propagated, and the remainders are destroyed.
If we project present trends into the future: genetic diversity will continue to decrease, the lack of sexual recombination will lower the potential for evolving pest resistance, and susceptibility to agricultural pests and diseases will continue to increase. When pathogenic organisms such as viruses, fungi, mites, and aphids attack a genetically uniform, asexually reproduced crop, losses are often extensive. Illegal drug Cannabis cultivation and breeding have historically suffered from similar problematic pest infestations.
Along with the unfavorable tendency to produce male flowers and unwanted seed, modern varieties have some other drawbacks: they are increasingly finicky about growing conditions, are susceptible to pests (e.g., various mites) and disease (e.g., gray mold and powdery mildew), and are generally difficult to grow. Increasing numbers of pests and diseases lead to increasing use of unapproved pesticides and plant growth regulators, which can directly impact public health and the health of surrounding pollinator & wildlife populations (songbirds etc.), ecosystems & watersheds.
Economic effects of genetic erosion in cannabis:
Commercial sinsemilla cultivars are asexually reproduced, and almost all share varying amounts of both Narrow Leaf Drug (aka “sativa”) and Broad Leaf Drug (aka “indica”) heritage:

Founder effects from Broad Leaf Drug landrace selections introduced gray mold and powdery mildew susceptibility that cause millions of dollars in crop losses annually: The major horticultural drawback to Narrow Leaf Drug x Broad Leaf Drug hybrids is their susceptibility to fungal infections: Their large, dense, tightly packed female inflorescences (commonly called “buds”) hold moisture and make perfect microclimates for bud rot caused by the gray mold (Botrytis cinerea Pers.), known to fine wine makers as the “noble rot” of grapes.
NLD/BLD cultivars have little natural resistance to fungal infection because the BLD founder plants were naturally adapted to the arid conditions of Afghanistan where pathogenic fungi cause little threat. Many NLD landraces originated from regions with relatively more humid conditions (Colombia, India, Jamaica, Thailand, etc.) and evolved a natural resistance to fungal infection. Bud rot was almost unheard of when only NLD varieties were grown, not so today…



Novel gene combinations still arise because the modern drug Cannabis gene pool is based on traditional landraces from geographical origins that represent two genetically distinct primary gene pools, Narrow Leaf Drug (aka “sativa”) and Broad Leaf Drug (aka “indica”). Whenever a seed is planted, it represents the sexual union of two parents, and creates an opportunity for new genetic combinations to appear. Asexually reproduced cuttings are genetic dead ends—only sexual reproduction offers us a truly brighter genetic future and the possibility for plant improvement.
When a Cannabis landrace is not reproduced every five to ten years, the stored seeds will most likely die and the landrace may be gone forever. Seeds must be properly kept in a gene bank (including on-farm living gene banks) and reproduced periodically under ideal conditions. The past 50 years have seen the genetic diversity of the Cannabis genome dwindle away. Indeed, the vast majority of landraces may already be extinct, and we therefore must be careful to preserve and multiply what remains. As Watson and Clarke warned us in 1997,
“Many local landrace varieties, the result of hundreds of years of selection for local use, have been lost because of Cannabis suppression and eradication, neglect on the part of agricultural officials and industry, anti-hemp propaganda and the general trend (until recently) to reduce industrial hemp breeding and research. Genetic materials are a living heritage and we are their custodians. We must concentrate our efforts to collect, preserve, characterize and utilize the remaining Cannabis genetic resources before it is too late.”
As the worldwide reduction in Cannabis diversity continues, the importance of genetic preservation becomes more obvious and critical. The Cannabis gene pool is amazingly diverse, but it needs our help as growers and consumers…
What is a landrace aka local ecotype?
There is not one official legal or regulatory definition of the term “landrace” nor has a widely accepted definition emerged, landraces have been defined in a variety of ways and the definition has evolved as our collective understanding has grown of landrace population genetics:
A definition which well describes the complexity of landraces, is that a landrace “is a dynamic continually-evolving population(s) of a cultivated and/or wild-gathered plant that has historical origin, distinct identity and lacks formal crop improvement, as well as being genetically diverse, locally adapted and associated with traditional farming systems”. More generally, landraces have been proposed as one of two types: autochthonous (indigenous/local) when cultivated for more than a century (100 years) in a specific region, or allochthonous if introduced in another region due to migration (known as seed flow).

Chthonic stems from the word autochthon, meaning “any of the earliest known dwellers in a region; an original inhabitant, an aboriginal” or “a being living in his or her place of origin” (The New Oxford English Dictionary).
Another definition: “A landrace of a seed-propagated crop can be defined as a variable population, which is identifiable and usually has a local name. It lacks ‘formal’ crop improvement, is characterized by a specific adaptation to the environmental conditions of the area of cultivation (i.e. tolerant to the biotic and abiotic stresses of that area) and is closely associated with the traditional uses, knowledge, habits, dialects, and celebrations of the people who developed and continue to grow it.”
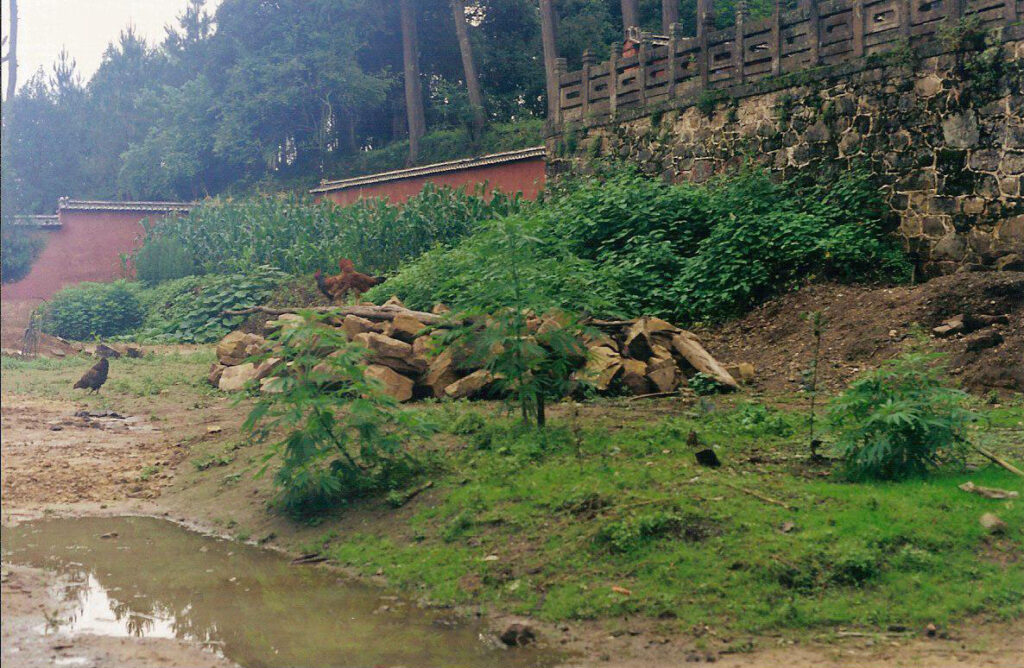
Landraces have been cultivated for many generations under adverse conditions in a particular region without conscious selection, and have become adapted to those adverse conditions unique to a particular locale.
Conditions for the origin of landraces include long-term cultivation (at least 100 years: 30 years is considered “localized” i.e. beginning to adapt to local conditions, after 100 years a variety is considered to be a locally-adapted/naturalized landrace) in a particular region under natural selection, which results in a stable appearance. Selection by man is mostly done unconsciously; nature selects for characters such as frost tolerance, drought tolerance, and low temperature adaptation.
A local ecotype is a subset of a species that has adapted to a specific geographic and ecological environment and as a result has evolved to be genetically distinct from other members of the same species found in different environments. Different ecotypes of the same species can usually form fertile hybrids.
An ecotype is a population (or subspecies or race) that is adapted to local environmental conditions. The implication is that those individuals which were best adapted to the prevailing conditions left the most offspring. Moreover, those more successful individuals carry genes that are partly responsible for their success in that environment. Thus, the adaptations of these ecotypes are based on the interactions of their own special sets of genes with their own environment.
Much of the observed variation in ecotypes results from the ability of individuals to respond differently to changes in environmental factors. All individuals have some range of response as the environment changes. For instance, plants from the same population usually grow taller in the longer growing season of low elevations than they do in the shorter growing season of high elevations. To separate the phenotypic and genotypic components of variation in ecotypes, it is necessary to grow representatives from each ecotype under the same conditions.
A classic example of ecotypic variation is the 1948 study of the ecotypic landraces of yarrow (Achillea lanulosa) across the Sierra Nevada. With rising elevation, winter temperature drops below freezing, so that winter dormancy is necessary and plants are smaller. On the eastern slope of the mountains, individuals flower late and are adapted to the colder, more arid climate.

Since the major attributes of these races were maintained when grown under uniform conditions in the same place, it is clear that these adaptations had a genetic basis. That is, each of the races had diverged genetically from the others in response to the challenges of their local environments.
Landrace / local ecotype populations have higher genetic diversity
Landrace varieties emerged in different regions of the world as a result of millennia of natural selection and evolution in a multitude of traditional local agrosystems, leading to heterogeneous (genetically-diverse) populations rather than a few superior genotypes. Farmers have been the keepers of diversity, mass-selecting genetic materials with desired traits and saving seeds for subsequent growing seasons and increasing gene flow through seed exchanges with relatives and neighbours or through the introduction of local or exotic materials. The exchange of seeds between farmers ensures the maintenance of the genetic heterogeneity (diversity) of the landraces, which can contribute to the creation of new local varieties (populations), as well as groups of interrelated local varieties (which could be considered meta-populations).
Landraces adapt to specific agro-climatic conditions while maintaining considerable genetic diversity/heterogeneity between and within populations, constituting a huge reservoir of genetic diversity that is interesting for future breeding work to improve important agronomic traits of crops such as resistance to biotic and/or abiotic stresses, thus sustaining yield and quality in increasingly unpredictable and unfavourable environments. Therefore, the exploration of landrace genetic diversity and conservation for future generations is important.
While landrace populations are variable, diversity is far from random. They consist of mixtures of genotypes all of which are reasonably well adapted to the region in which they evolved but which differ in detail as to specific adaptations to particular conditions within the environment. They differ in reaction to diseases and pests, some lines being resistant or tolerant to certain races of pathogens and some to other races. This is a fairly effective defense against serious epiphytotics. Some components of the population are susceptible to prevalent pathogenic races, but not all, and no particular race of pathogen is likely to build up to epiphytotic (catastrophic) proportions because there are always resistant plants in the populations. Landraces can be lower yielding (vs. modern cultivars) but more dependable/yield-stable. They are adapted to the rather crude land preparation, seeding, weeding and harvesting procedures of traditional agriculture. They are also adapted to low soil fertility; they are not very demanding.
When landrace populations are maintained, between- and within-landrace diversity continues to evolve because of the natural and human selective pressures. However selection does not lead to genetic uniformity and each landrace in itself is highly genetically diverse (i.e. a mix of different genotypes constitute a landrace). Among- and within- landrace diversity had been the key to agriculture’s food security for generations. The mix of landrace diversity has allowed (and still allows) farmers to service a diversity of needs and purposes and to obtain a harvest whether the year is dry or wet, hot or cold, or whether there is a pest or disease attack, as some genotypes will be affected each year but not all of them.

Landraces have a certain genetic integrity. They are recognizable morphologically; farmers have names for them and different landraces are understood to differ in adaptation to soil type, time of seeding, date of maturity, height, nutritive value, use and other properties. Most important, they are genetically diverse. Such balanced populations – variable, in equilibrium with both environment and pathogens, and genetically dynamic – are our heritage from past generations of cultivators. They are the result of millennia of natural and artificial selections and are the basic resources upon which future plant breeding must depend.
Landraces differ from the cultivars developed by modern scientific plant breeding in that they have not been deliberately intensively selected to a predetermined reduced level of genetic heterogeneity.
Many landraces could be considered as local ecotypes, i.e. a landrace is a local or ecological race with genotypes adapted to a particular restricted habitat as a result of natural selection within the local environment.
“an autochthonous (indigenous/native) landrace is a landrace variety grown for a long period in the farming system concerned with a high capacity to tolerate biotic and abiotic stress resulting in a high yield stability and an intermediate yield level under a low input agricultural system. As the environment changes annually and as the landrace becomes ‘contaminated’ with few genotypes of other landrace(s), or cultivar(s) it will continuously adapt itself ”
Landraces are continually evolving and also continually mixing with other landraces or cultivars on a genetic level. Natural and artificial selection together with migration resulting from the exchange of seeds can contribute to creating different landraces (populations), as well as groups of interrelated landraces (metapopulations). Therefore, landraces should be viewed as evolving entities in contrast to modern cultivars, which are expected to be maintained true to type:
Phenotypic plasticity is “the ability of individual genotypes to alter their growth and development in response to changes in environmental factors”. It is flexibility of response and allows a population to survive in a broad range of environments, especially marginal conditions. It is a key component of the genetic system of weeds and is often critical to the ability of species to diversify and adapt in response to natural and human-caused selection.
By virtues of several of its ecological adaptations, Cannabis has exceptional adaptive phenotypic plasticity.
These adaptations include: ability to grow large and produce many seeds in ideal environments or to survive as a dwarf and produce at least a few seeds in very inhospitable circumstances; roots develop as a widespreading fibrous system near the surface, where deep soils are too waterlogged, or grow very deep as a taproot to find water in dry soils; damaged main stalks regrow abundantly from lower branches; and tolerance of shade, despite being primarily a full-sun plant.
Landraces represent the most ancient pure form of cannabis we have available, perfected by Mother Nature over hundreds, sometimes thousands of years, constantly adapting and evolving, improving their harmony with the environment they live in.
Landrace plants are larger and generally more vigorous; buds usually form more complex resin that contains more cannabinoids and terpenes. If allowed to develop large in size, these seeds and plants can give massive yields. Perhaps most importantly, landraces are the basis for breeding and necessary to creating new and different strains of cannabis.
Arjan (the strainhunter) always says, “If you are looking for cannabis, find man first,” because man uses cannabis and man propagates cannabis the most, since the beginning of our existence. In most cases the real landraces are found within poor, isolated rural communities in remote areas of third world countries. Unfortunately, many landraces are at risk of extinction because of eradication programs or crop-replacement government programs, as has happened in Jamaica and Trinidad.
Mixing genes is always a good thing for the development of any species. This is one of the basic rules of genetics, valid for all forms of complex life on this planet. The thousands of strains available today are the result of over 40 years of incestuous breeding in the western world. Modern cannabis breeding as we know it began on the West Coast of the United States and Canada in the mid-1960s—and hasn’t stopped. It just kept spreading and increasing exponentially. However, there is a need for injecting new genes into the mix. And the best “new” genes are “old” landraces, for the simple reason that they have been outcrossing and naturally selecting and adapting themselves on a massive scale for long periods of time.
Cannabis hemp is a very complex plant: unlike most plants, it’s out-crossing, with male and female plants (aka dioecious). So it’s nearly impossible to self an individual for a trait that’s favorable – you can do it artificially but it’s not the natural situation.
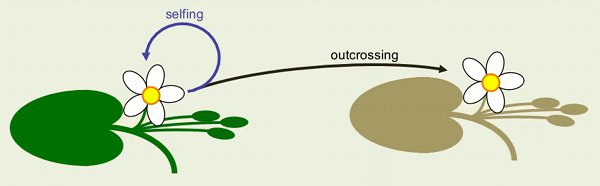
Plus cannabis is also wind-pollinated, so unless you isolate plants to keep them from being pollinated by other ones in the neighborhood, then the pollen blows around in the wind, so one parent, one plant can be a parent to many, many offspring with very many mates. So this is also making for diversity constantly in cannabis. And we, as breeders, fight this diversity by trying to narrow it down to a variety that breeds relatively true. But this is a difficult path. It’s easy to make cannabis seeds, but it’s not an easy plant to breed in a structured way that leads to plant improvement, leads to better varieties.
Cannabis’s natural history is that it wants to be an out-crosser, it’s always striving for variety in its own survival mechanism, if you will. And as breeders we’re trying to narrow that variety so that a cultivar will have certain traits: mature in a certain number of weeks, have a certain aroma, and a certain cannabinoid content. It’s always a battle between having enough diversity that the plant is genetically healthy and not terribly inbred, but inbred enough so that it’s not just a dog’s breakfast of different types in one population.
Key differences of landraces vs. cultivars: yield stability & resilience adaptation
A landrace should not be compared with a cultivar, because yield stability is the major characteristic of a landrace, whereas a high yielding capacity under optimal improved cultivation conditions characterises a cultivar.
Yield stability of landraces under traditional low input agricultural systems is due to the fact that whatever the varying biotic and abiotic stress for each plant, due to the diverse gene pool (large number of genotypes) one or more genotypes within the landrace population will yield satisfactorily. Landraces were and still are grown by farmers, market and private gardeners all over the world for this reason.

Landraces have a high ‘stability of their characteristics’ and great resistance capacity to tolerate adverse influences and stressors. Their production capacity in an optimal modern controlled cultivation environment, however, can sometimes be less than that of cultivars and when grown outside their home region, their genetic composition will change.
However in more marginal environments or during times of environmental stress, landraces can yield more than cultivars: In marginal environments, many traditional varieties out-perform modern cultivars in drought resistance, field adaptation, flood resistance, or resistance to weeds, disease, and insects. In highly productive environments, landraces have also been shown to outperform high-yield cultivars. (For example, in a village in Central Java/Indonesia, farmers collected 26 traditional rice varieties and compared their performance with government-provided high-yield cultivars and discovered that, absent of chemical inputs, seven of the traditional varieties outperformed three high-yield cultivars on total yield per hectare.)
Genetic diversity within a farming system also provides more crop stability in terms of yield security and encourages more sustainable production methods, because the dependency on outside-farm inputs is much lower…
Many traditional societies have developed landrace-based polycultures that are capable of providing a higher yield and increased land-use efficiency from a given area than from an equivalent area sown in separate patches of monoculture (for example, one hectare of sorghum + pigeon pea mixture produces the equivalent of 1.62 hectares of sorghum monoculture + pigeon pea monoculture. Increased land use efficiency has been reported for other polycultures including millet + groundnut, maize + beans, millet + sorghum, maize + pigeon pea, maize + cocoyam + sweet potato, cassava + maize + groundnut. Modern industrial “Green Revolution” agriculture methods actually reduced food yields in these cases…)
Landraces differ from non-landraces in a number of traits which together appear to form an adaptive complex. Moreover, within a landrace these traits are not just present in only one combination, but in different combinations in different individuals. For each site and for each year the composition of the population becomes adapted to the conditions of that site and that year.
Landraces adapt better to environmental stresses & pressures:
Landraces, thanks to their long evolutionary history of continual adaptation to stressful environments, are ideal genetic resources to explore novel genetic variation for responses to environmental constraints.
In particular, landraces are an effective source of useful alleles (allele = a particular combination/ version of a DNA sequence occurring at a fixed known point on a chromosome, each allele provides the genetic code for a particular trait/quality) to sustain yield and quality in both favourable and limiting environments. In some cases, the gene loci involved in the control of yield in good conditions can still maintain a good level of production when stresses are mild. In other cases, alleles which specifically express in environments with more pronounced stress conditions have been identified in landraces, which can help in breeding for improved lines well adapted to specific areas.
Landraces may also carry new sources of pest, disease and pathogen resistance that can be exploited to enrich the narrow resistance spectrum currently found in adapted cultivars.
Crops grown in many areas worldwide, for example the Mediterranean area, are often exposed to biotic and abiotic stresses such as high temperatures and water stress. Compared to modern cultivars, landraces are often more resilient to stresses and represent a valuable source of germplasm for meeting the future needs of sustainable agriculture in the context of climate change and ecosystem change.
The wide genetic variability and high potential for adaptation to extreme climatic conditions of landraces make them important for genetic improvement, particularly in improving agronomic traits such as yield and yield stability, the wide genetic variability also confers a broad range of traits that can help a plant/organism adapt to various environmental stresses & pressures.

Examples of environmental adaptation traits found in landrace populations include:
Submergence tolerance trait/gene (locus SUBMERGENCE 1) (confers superior performance in waterlogged environments) has been identified in some landrace populations.
So has a gene for drought-tolerance.
A gene/trait has been found which confers salt/salinity-tolerance (the SKC-1 gene encodes a sodium transporter that controls K+/Na+ homeostasis under salt stress)
Another trait potentially limiting crop production is boron toxicity. Tolerance to toxicity is associated with the ability to maintain low boron concentrations in the shoot and has been found in multiple crop species: The Bot1 gene (in barley landraces) is responsible for the high boron-toxicity tolerance of the Algerian barley landrace, as is the boron toxicity gene Bo4 in boron-tolerant bread wheat landraces.
Crop genotypes have been found that have higher productivity under low-nitrogen conditions: “An important trait we are particularly interested in is nitrogen-use efficiency, there are economic and environmental benefits of reducing nitrogen, and we really have not tried to do this in the last century. Most of the breeding effort has been to push yield up under high-nitrogen conditions. When we did this, we lost some of the good genes for productivity under low-nitrogen conditions… There is still a lot of natural variation in the gene pool that can be optimized. For a lot of the sustainability traits like drought and nitrogen tolerance and pest resistance, nature does give us what we need; we just have to find it.”
As regards phosphorus deficiency, widespread in tropical soils, the well-known gene Phosphate uptake 1 (Pup1) increases phosphorus uptake and confers significant grain yield advantage in phosphorus deficient soils. Pup1 is found in landraces or cultivars adapted to drought-prone environments and is effective in different genetic backgrounds and environments.
Another phosporus-adapation gene is POSTL1 which significantly enhances grain yield in phosphorus deficient soils, promoting early root growth, thereby enabling plants to acquire more phosphorus and other nutrients.
An allele (gene combination) has been found which increases ground coverage after winter, which could be exploited as an adaptive trait in some environments.
Landrace alleles have been found which contribute to an acceleration of flowering, increase in thousand-kernel weight and increase in plant height.
Landraces are also known to adapt/form based on elevation: in Chiapas (Mexico), three corn/maize landraces make up 90 percent of all the maize farmed: the Oloton–highland variant, the Comiteco–midelevation, and the Tuxpeño–lowlands landraces. These landraces, which also have sub-varieties within their respective groups, have adapted over time to their local environments with distinct phenotypes. The higher up you go, the more the landraces predominate on the farms.
Landraces are part of culture and lifestyle for Chiapans: Chiapans, like other Mexicans, grow because “it is more about something they feel they need to do that is part of their way of being.” The cultural identity of many groups is tied to essential ingredients (i.e. crops) of their local cuisine – for example, 15 different dishes in Mexican cuisine have been identified that require 15 different traditional varieties of maize…
The landrace varieties are being investigated to their responses to climate change pressures: the local landraces are adapted to very restricted regions and climate change could cause disequilibrium/ disruption in farming systems. University ecologists and students are planting different landrace varieties at different altitudes, checking for adaptation resiliency. “When we cross highland landraces with midelevation or lowland varieties, the cross is better than original varieties when planted in foreign environments.. These seeds are the product of an uninterrupted evolutionary process and are something very valuable. Without the landraces, we are not going to be able to look for genetic resources that can help crops adapt to the challenges that agriculture is going to go through, especially how our crops are going to cope with changing climate.
We are trying to better understand the genetic mechanisms of landrace stress responses and to share our knowledge so that what we learn is used for everyone’s benefit. I would like to see us all—both the scientific community and the public —spend our time on important questions whose answers matter for a sustainable Earth system.”
Environmental adaptations across the major cannabis hemp gene pools:
The ecological species concept defines an ecotype as a population of organisms adapted to a niche (a set of resources aka a particular combination of environmental variables/conditions, stressors & constraints). Ecology drives evolution, the ecological niche that a plant population occupies will shape its morphology and phytochemistry. Ecological niches give rise to reproductive isolation barriers, such as the habitat isolation between the two primary cannabis gene pools: C. indica (in warm-and-wet India/southeast Asia) and C. afghanica (in cool-and-dry Afghanistan):
Temporal isolation between the major cannabis gene pools arises in the form of separate flowering times, such as the temporal isolation observed between wild-type C. ruderalis and neighboring C. sativa: Wild-type plants matured in mid-June, while domesticated plants were still in the vegetative stage. Temporal isolation has thwarted attempts to cross German C. sativa with South Asian (India) C. indica: By the time Indian males produced pollen, German females had already passed their fertility period. When attempting to pollinate Indian females with German pollen, frost killed Indian females in October before they could set seed.
Habitat isolation arises in the form of genetic fitness for a specific environment. Habitat isolation can be tested in transplantation experiments: C. himalayensis adapted to the Himalaya (high altitude, cooler) may not survive when transplanted to the plains of India (low-altitude, hotter). C. indica adapted to the warm-and-wet plains of India would likely succumb in the Hindu Kush, with its arid climate, desiccating winds, high UV-B radiation, and shorter growing period. C. afghanica is poorly adapted to warm-and-wet conditions—the seedlings are susceptible to lethal diseases caused by Pythium and Rhizoctonia fungi in damp soil. In mature plants, roots suffer waterlogging stress, branches snap under monsoonal rainfall, and flowers perish from “bud rot” caused by Botrytis cinerea and Trichothecium roseum. Backcross experiments show that intolerance to humidity is expressed in hybrids that contain a small percentage of C. afghanica parentage. And, tropical landraces may not reach maturity in studies conducted outdoors at temperate latitudes. Frost kills them first.


Chinese hemp (Cannabis chinensis) expresses a wider branch angle than European hemp (first observed in 1874). Branch angle may be a function of internode length: the long internodes of “Sativa” allow development towards overhead sunlight, while the short internodes of “Indica” force the branches to grow laterally.
Branch laxity is a qualitative measure of flexibility, the ability of a branch to bend or droop. Compare branch laxity in weeping willow (Salix babylonica) to that in Scots pine (Pinus sylvestris). Laxity likely reflects the ratio of bast fiber (flexible) to wood fiber (inflexible). Cannabis plants from the Himalayas tend to be more laxly branched than plants from the Indian plains, with branching in Afghanistan plants being the least lax. And branches are more lax in Chinese hemp than European hemp.
All commercial hemp cultivars are presently adapted to growing at or above 45 degrees latitude, and will yield less fiber when they are relocated as little as two degrees closer to the equator. Closer to the equator, the day length is shorter earlier in the summer, and consequently the Cannabis crop will flower prematurely when plants are small, which lowers fiber and seed yield. European hemp cultivars cultivated closer to the equator than within their normal latitudinal farming range are also attacked by a host of alien pests and diseases for which they have little resistance. As a result, no hemp varieties approved by the EU can (as of yet) be successfully grown closer to the equator than at about 40 degrees latitude. Limited traditional hemp production in other regions of the world relies mostly on unimproved local landraces.
Differences in traits between wild-type vs. cultivated/ domesticated cannabis
When considering landraces it is worth noting the distinct differences in traits and growth characteristics between cultivated cannabis and wild cannabis; one can recognize a syndrome of characteristics that differentiate wild C. sativa from domesticated kinds of fiber hemp, oilseed hemp, and marijuana plants:
Taxonomists have long (since at least the 1840s) recognized three population types for Cannabis based on natural origins and associations with humans: those that are truly wild, those that are cultivated, and feral escapes from cultivation that grow spontaneously in areas associated with and often disturbed by humans:
Wild-type cannabis plants are branchier than domesticated plants, and the leaves smaller. Wild-type plants mature earlier than domesticated plants; tend to be more drought tolerant, and tolerate shade better. Achenes (seeds) shatter from plants, and their germination is slow and uneven. Cultivated varieties grow to a greater height and thickness of the stem, producing a heavier and fuller seed, and not throwing out so many branches.
Gland heads of wild-type Afghani plants will abscise (separate from the main body of the plant) after a hard rain, whereas they remain attached in cultivated South Asian C. indica. This may reflect natural selection—Autumn rains are rare in Afghanistan, compared to monsoonal South Asia. Artificial selection may have also played a part—freely abscising gland heads are favorable for producing sieved hashīsh. In South Asia, where gañjā is consumed, persistent gland heads are favorable.
Cannabis landraces and cultivated varieties, in general, also have larger and lighter colored seeds than wild or feral populations growing nearby; in addition, the seeds of landraces also generally lack the horseshoe-shaped base and mosaic-patterned perianth associated with freely-shattering, camouflaged, wild-type seeds. The East Asian Broad Leaf Hemp gene pool exhibits the greatest diversity in seed coloration and size, resulting from millennia of natural and human selection within a large geographically and culturally diverse region. Chinese wild and feral seeds range in size from 100 to 500/g (approximately 2800 to 14,000/ounce), whereas large snack food seeds might have as few as 15/g (approximately 420/ounce), representing more than a twenty-fold range in seed size.


Changes in seed physiology also accompany domestication. For example, mechanisms for perennation (inhibition of seed germination that allows seeds to survive through winter before germination occurs in the spring) are often lost. Cannabis farmers traditionally collect seeds and store them, ensuring their survival until the following spring, and, as a result, perennation is no longer of natural evolutionary advantage.
Seeds of naturally selected feral populations evolved in response to climate fluctuations and germinate slowly and unevenly, whereas cultivar seeds are adapted to agricultural norms and must germinate quickly and uniformly. Densely sown hemp field conditions automatically select for uniform and rapid germination because late-germinating seedlings cannot compete with already established seedlings and are crowded out, thus allowing for more uniform treatment of the crop. Cultivars that have lost their dehiscence mechanisms, as well as physiological means of delaying germination, depend upon human intervention to perpetuate them—a strong sign of domestication.
Domesticated Cannabis easily escapes cultivation and goes “feral.” Cannabis, like cats, is barely domesticated. As a crop plant, the species is rather unique in this regard—relatively few crops can thrive outside of cultivation, cannabis is a notable exception. Domesticated C. sativa reverted to a wild-type phenotype in Canada just 50 generations (years) after cultivation was prohibited.
This rapid phenotypic evolution makes it difficult to distinguish truly wild plants from formerly cultivated plants that have reverted to wild-type phenotypes. Ecologists have delineated a spectrum of plants that exhibit wild-type traits, ranging from truly wild (i.e., native, indigenous, aboriginal), ruderal (either wild or weedy), naturalized (i.e., weedy), to spontaneous (escapes of cultivated plants).
Cannabinoid ratios are another factor that varies between wild and cultivated cannabis: The vast majority of wild hemp and hashish populations contain approximately equal amounts of CBD and THC (aka Type II, with THC: CBD % = ~1:1), which, in the absence of human selection, may be the naturally selected and evolutionarily neutral character state of major cannabinoid production. Within intermediate Type II (THC:CBD % = 1:1) landrace populations, seeds selected from the lowest THC:CBD ratio plants, as well as those seeds selected from the highest THC:CBD ratio plants, when sown in isolated gardens, will produce offspring populations with median cannabinoid ratios skewed in favor of either the high-THC (Type I) or high- CBD (Type III) parents.
When these selection processes are repeated for a few generations, the following two distinct chemotypes or chemical phenotypes result: one with a narrower range of cannabinoid ratios and higher average THC content (Type I); and a second with a narrower range of cannabinoid ratios and higher average CBD content (Type III).

Since psychoactive THC and terpenes are easily detected by simple human assay, early Cannabis farmers readily developed more potent landraces by annually selecting higher potency plants and sowing their seeds. Traditional Asian NLD (narrow-leaf drug variety) landrace farmers from Thailand, India, and South Africa, as well as New World farmers in Mexico, Colombia, and Jamaica, maintained varietal purity by sampling individual plants and selecting seeds from the most flavorful and potent ones to sow the following year.
All Cannabis plants are wind-pollinated, which allows them to intercross freely; mass selections or phenotypic selections are usually made by choosing the best plants in the field before harvest, and saving their seeds for sowing. Both mass family and single plant selections are made to produce more genetically homogenous lines for breeding.
This is essentially how local feral populations become landraces: Individual female plants are selected, but a single female plant is usually fertilized by a random assortment of many unselected male plants, and, as a result, most of the offspring are half siblings, rather than full siblings.
Domestication and the loss of wild-type traits is a product of human selection, which began unconsciously (selecting plants with seeds that did not shatter).
In addition to the features described above, humans have selected seed-bearing crops that mature most of their seeds more or less simultaneously, to minimize harvest loss. As well, a highly congested fruit axis (adjacent seeds very close together along with bracts and young leaves) makes it very difficult for seeds to fall away from the plant and facilitates harvest of the seeds. A corn cob illustrates just how close together seeds can be packed and how most of them mature more or less simultaneously. These features are evident in plants selected for yield of seeds. By contrast, wild C. sativa plants mature seeds sequentially over a long season, and the seeds are relatively well separated so that they do not interfere with each other’s ability to fall off the plant.
In contrast to domesticated seeds, wild seeds have a comparatively thick fruit wall or “hull.” This (1) protects the seeds against mechanical abrasion; (2) makes it more difficult for herbivores to break open the seeds and consume the embryos; (3) makes it more difficult for water to penetrate, so that the seeds won’t germinate and will remain dormant, providing for germination over several years; and (4) keeps oxygen from entering and degrading the storage oil. Possibly, the thick shell also serves to protect the seeds from being digested while they are in the gut of some animals, so that they will be deposited and germinate in the animal’s excrement, an environment to which cannabis is adapted.
A camouflagic mottled layer covers the seeds of wild C. sativa, providing some protection for the fallen seeds against mammalian and insect herbivores. The layer is developmentally homologous with the perianth—the petals and sepals of many flowers. The dark appearance of wild seeds also contributes to their being inconspicuous. By contrast, light-colored seeds have often been selected in cultivated plants. Wide Leaf Drug biotypes (i.e., Central Asian landraces) tend to express pigmented cells in linear stripes, whereas Narrow Leaf Drug biotypes (i.e., South Asian landraces) express pigmented cells in irregular mottling. This character may not have taxonomic relevance; Crescini (1940) showed pattern variations within a single landrace (Carmagnola).

Unlike the seeds of cultivated varieties of C. sativa, wild seeds of the species are generally at least somewhat dormant and germinate irregularly, features that adapt the plants to the environmental fluctuations typical of wild habitats. The dormancy requirement is typically satisfied by a period of cold treatment, aging, or some other natural condition furnished by nature. For example, some fully mature Kansas wild hemp seeds could be germinated in the laboratory within three weeks of maturity in the fall, while seeds stored at room temperature reached maximum germinability in three months, but seeds stored at 5°C reached maximum germinability only after five months (immature seeds, if viable, required a considerable period before they could be germinated).
And a 1924 study of Russian wild hemp seeds showed that fewer than 10% could be germinated immediately after maturation, but that repeated watering and drying cycles increased germination (water-soluble germination inhibitors may be present in wild hemp), the germination of Russian wild hemp proceeds slowly and intermittently.
Although seeds of C. sativa will germinate in the light or in darkness, it’s been found that light partially inhibits the germination of wild hemp. This may represent a natural adaptation for seeds to await the darkness of being buried by soil before germination. Wild (feral) seeds germinate better after a period of storage at cold temperatures, while domesticated seeds germinate quickly on being watered, reflecting adaptation to remaining dormant overwinter by wild seeds. Clearly, wild seeds benefit from a period of cold stratification to overcome germination inhibitors. Wild seeds in northern areas germinate at lower temperatures in the spring than the seeds of domesticated plants, indicating adaptation to the cold spring temperatures of north temperature climates.
Selecting mutations that inactivate the separation mechanism (abscission, i.e., breaking away of fruits at their base so they fall away) or the dehiscence mode (i.e., opening of fruits to release seeds) with the result that the mature seeds or fruits remain on the plant greatly facilitates harvest. This reduction of “shattering” (natural shedding of seeds at maturity) is the most important way that humans have domesticated the majority of crops.
Wild cannabis hemp ecology
The various traits of Cannabis recorded in the literature have been collated and correlated with traits adapted to specific biomes (biome = a distinct biological community that has formed in response to a shared physical climate). Based on these correlations, Cannabis is now thought to have evolved in a steppe biome at a temperate latitude, in undulating terrain that was cut by seasonally-flooding streams or rivers, populated by herbivores.
The ecological requirements and genetic inheritance of plants determine where they grow naturally. Cannabis plants require well-drained soils, adequate sunlight, warmth, and moisture, so most naturally growing populations are found seasonally across accommodating northern temperate latitudes.
Under natural conditions, Cannabis grows well along exposed riverbanks, lakesides, the margins of agricultural land, and other areas disturbed by humans:
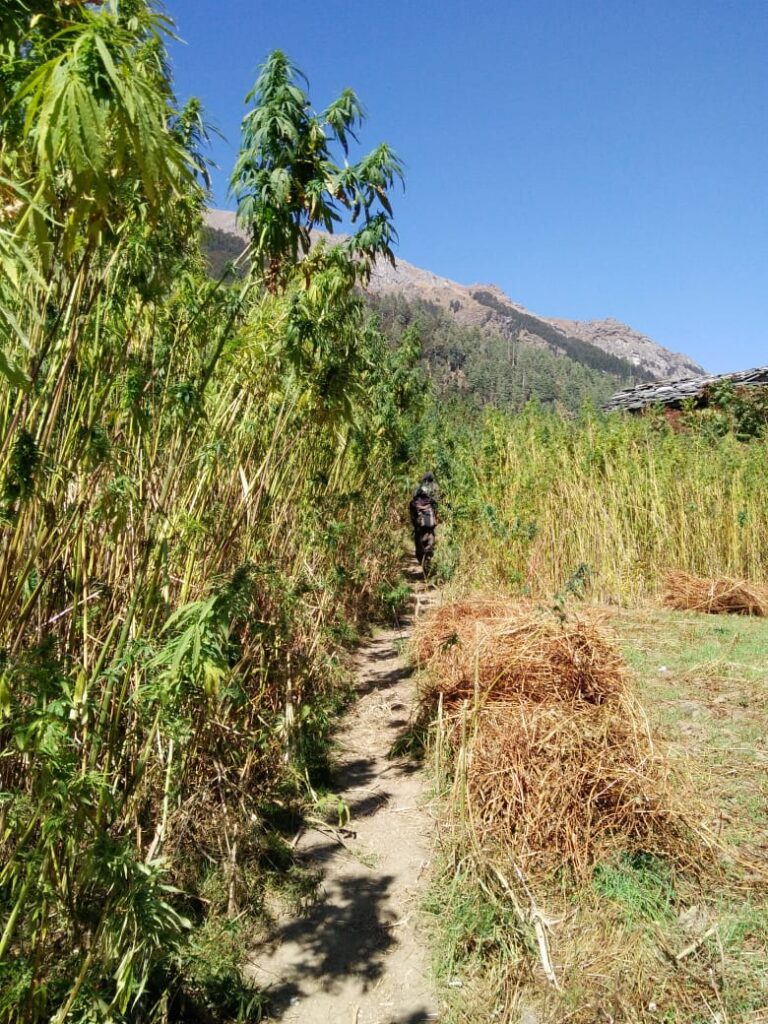
As a weed in North America, C. sativa occurs in farmyards, waste places, vacant lots, in disturbed areas of pastures, occasionally in fallow fields, and along or beside roadsides, railways, ditches, creeks, fence rows, borders of cultivated fields, bridge embankments, lowland drainage tributaries, and open woods.
The species seems very poorly adapted to penetrating into established stands of perennial vegetation and generally invades such areas only after the soil is freshly disturbed. As a colonizer, weedy hemp spreads slowly, except in drainage channels (the appellation “ditch weed” reflects its preadaptation to moist depressions). Weedy hemp in the United States is often found in alluvial sites disturbed by flooding. In Illinois it was found that disturbance of the soil was extremely important in determining whether or not C. sativa would establish, and moreover, the less fertile the site, the more hemp was dependent upon soil disturbance.
Wild hemp populations in Illinois do not persist at a given site unless continuously disturbed, but may remain in a given area provided nearby disturbed sites are regularly generated. Nearly all the populations studied were on sites repeatedly disturbed by cultural activities, such as mowing, cultivation of soil, stream channel improvements, and refuse disposal. Ruderal/wild hemp appears to be progressively more restricted to optimum and disturbed sites toward the ecological limits of its range. A 1972 survey of 1700 wild hemp stands in Kansas showed that 94% of the stands were associated with such past soil disturbances as construction, land clearing, tillage, livestock trampling, or flooding. Wild hemp was found in borders of cultivated fields (primarily fence rows), on nonagricultural land (primarily right-of-ways and bridge embankments), native pastures, abandoned fields, cultivated pastures, and in cultivated fields. Wherever it is found, ruderal C. sativa usually associates with other weeds, as one would expect from the habitats and sites described here.
Cannabis sativa grows best on sandy loams. Because it is very intolerant of waterlogged soils, weedy hemp seldom survives on soils high in clay that retain water. For example, weedy hemp in Illinois appeared to tolerate many soils (but not those with more than 40% clay), provided that the roots receive adequate aeration. Well-drained bottom land is particularly attractive to ruderal hemp, and is often found near streams and creeks.

Nitrogen is the element that most plants in nature find to be so deficient in availability that growth is limited. As expected, therefore, most of the world’s plant species are adapted to substrates in which nitrogen is in short supply, and very few are nitrophiles (nitrogen-loving). Plants classified as nitrophiles strip soils readily of nitrogen and are dependent on high levels of available nitrogen in the substrate. Wild C. sativa is a nitrophile, growing lushly in nitrogen-rich habitats such as well-manured sites, but growing very poorly when the element is deficient. Indeed, one of the dependable indicators of the possible occurrence of weedy C. sativa is the presence of nearby manure or livestock. In 1926 it was noted that wild hemp in Russia thrives in low places and ravines into which wild animal excrement is washed and on soils fertilized by grazing cattle. Manure not only supplies nutrients, but also the humus is important in retaining moisture that hemp demands. Weedy hemp is often found on the margins of crop fields, where the plants can take advantage of fertilizer run-off.
Hybrids of C. indica and C. afghanica could have arisen naturally. Their ranges are not far apart, and migratory animals could have carried seeds from one area to the other. For example, the Himalayan Greenfinch (Hypacanthis spinoides), whose “favourite food is the seed of the wild hemp,” ranges from the Samana Mountains (on the border of Afghanistan) to Manipur in northeastern India. A 2004 study counted 793 seeds of Cannabis sativa in the crop of an Oriental turtledove, Streptopelia orientalis, caught on the border of Russia and Kazakhstan. S. orientalis is migratory, and its range includes northern India, Afghanistan, Turkestan, and China. Achenes (seeds) at both sites showed a mixture wild-type and domesticated traits.
Natural distribution/dissemination methods for cannabis hemp seeds
A variety of animals could serve to distribute the seeds of Cannabis in nature: Humans, other mammals, birds, and insects have been proposed as biotic disseminating vectors for wild hemp, and water movement may also be important. Since the plant often grows in hilly and submontane habitats, gravity could also play a role. Because wild C. sativa is dioecious, the most effective dispersal agents should distribute at least a seed of each sex to a given site, although pollen is distributed so widely that even isolated plants may participate in reproduction.
Since birds are strongly attracted to the seeds, they are likely the most important wild animals distributing them in North America. Birds are usually observed consuming seeds on cannabis plants but are much less frequently seen on the ground in pursuit of fallen seeds. Darwin observed (in 1859) that “Some seeds of hemp germinated after having been from twelve to twenty-one hours in the stomachs of different birds of prey.” Presumably the only hemp seeds that could germinate after passing through the digestive tract of birds were not macerated by the beak or gizzard. A 1972 study fed wild hemp seeds to upland game birds, noting that quail passed approximately one viable seed/700 seeds consumed, and doves passed one viable seed/12,400 seeds consumed. It is also possible that some seeds are transmitted by adhesion to claws or bills.

Hemp seed is a superb bird feed, and not surprisingly, wild bird feed has frequently been the cause of plants becoming established in a locality. In recent years, most jurisdictions in Western countries have insisted that hemp seeds be steam sterilized. However, sometimes, a small percentage (much less than 1%) of such sterilized hemp seeds in commercial bird feed can germinate, indicating that hemp seed in current bird feed may still be a continuing source of spontaneous plants.
Water: Weedy hemp in North America is often found in alluvial sites disturbed by flooding, and flood waters may serve to distribute the seeds by water transport. Hemp seed has been widely used as fish bait in fresh waters, and this too could result in distribution and establishment. Fish are known to distribute some seeds, but whether this is the case for Cannabis has not been investigated.
Mammals: Ruderal hemp clearly depends heavily on human activities for dispersal. Large wild herds of mammalian grazers probably were important to providing manured habitats for Cannabis, and the species characteristically grows in moist areas, so the mammals may have distributed seeds caught up in mud on their hooves. In more recent times, domestic livestock may similarly serve as distribution vectors. Small rodents are attracted to the seeds of C. sativa and may play a role in their dispersal. The extent to which the seeds of Cannabis can survive a journey through the digestive system of mammals remains to be examined, but since some seeds will survive the digestive tract of birds, the same is likely true for mammals.
Insects: Insects, especially ants, commonly drag seeds of certain plants to their nests in order to consume a fleshy edible portion that these plants supply on the outer part of the seed. Plants often produce such an “elaiosome,” i.e., a fleshy edible appendage on seeds or fruits serving to attract dispersal vectors, constituting an adaptation for distribution of the seeds. A 1924 study discovered such a symbiotic relationship between wild hemp and the red bug Pyrrhocoris apterus L, which was observed feeding on the attenuated base (attachment area) of the hemp seed, and concluded that the base was an elaiosome (adaptation to attract seed dispersal vectors/agents). Pyrrhocoris apterus (redbug) is widely distributed in Europe and Asia, thus it’s been speculated that the wide distribution of ruderal hemp was in part due to the insect. However, the insect is a generalized feeder that has no fidelity to Cannabis, and the bases of wild achenes/seeds of Cannabis and its relative Humulus do not develop genuine elaiosomes, although the detachment zone of the achenes is a weak area of the protective pericarp and might offer some limited nutrition to insects. Whether insects are significant dispersers of the seeds of Cannabis is unclear, but it is possible.
Conserving, protecting & regenerating landrace genetic diversity
The astonishing diversity of the cannabis gene pool is a precious living heritage and we are its custodians. We must concentrate our efforts to collect, preserve, characterize and utilize the remaining Cannabis genetic resources before it is too late.
As the worldwide reduction in Cannabis diversity continues, the importance of genetic preservation becomes more obvious and critical. The Cannabis gene pool is amazingly diverse, but it needs our help as growers and consumers:
In the words of the Hopi elders,
“Now is the 11th hour…
now is the time to act!”

Conservationists use two main approaches to conserve & preserve genetic material: ex situ and in situ. Ex situ is the conservation of genetic material outside of its natural habitat, for example in seed banks & gene banks. While in situ conservation is much preferable for crop plants, due to the precarious continued existence of unaltered aboriginal wild populations of Cannabis, preservation in seed banks (ex situ) is also an immediate priority.
In situ conservation (the preferred method) is carried out in “the natural habitats and includes the maintenance and recovery of viable populations of species in their natural surroundings” which includes both wild/uncultivated habitats and also cultivation on farms.
The in situ approach has two main approaches/techniques: (1) the genetic reserve, which is the natural long-term conservation sites of wild populations in their places of origin and (2) on-farm conservation, which is the sustainable management of genetic diversity (cultivars, wild and weedy species) in locally traditional agricultural systems. The advantage of using both in situ conservation approaches, especially on-farm, is to conserve the widest variability in gene pools within species, providing a natural laboratory for the continued evolution of traits (i.e., adaptive response to climate change).
Conservation biologists now recognize that a species must compete and evolve to have a viable future. Species do not exist in isolation, but as part of a larger ecological community. The community includes climate, soil, pollinators, dispersal agents, symbiotic species, competitors, pests, and pathogens. Species preservation efforts are now directed towards preservation of communities and habitats. In situ conservation is being given higher priority and the conservation focus is shifting back to the farm.
In situ conservation allows the continued evolution of plant characteristics. This is particularly important in regions susceptible to drought or other environmental extremes. It is under the conditions of environmental extremes that adaptation to stress occurs. And local adaptation plays a critical role in generating genetic differentiation amongst populations. The genetic diversity we know today evolved in varied and heterogeneous environments, in order to conserve genotypes we need to conserve a range of environmental conditions.
Genetic reserves are designated for wild species (such as crop wild relatives) and are defined as “the location, management and monitoring of genetic diversity in natural wild populations within defined areas designated for active, long-term conservation”. This involves the location, designation, management and monitoring of genetic diversity at a particular location (sanctuary/habitat reserve). The site is actively managed, even if that active management only involves regular monitoring of the target taxa, and conservation is long term, because significant resources will have been invested to establish the genetic reserve. This technique is the most appropriate for the bulk of crop wild relative species.



On-farm targets landrace conservation and is defined as “the sustainable management of genetic diversity of locally developed crop varieties (landraces), with associated wild and weedy species or forms, by farmers within traditional agricultural, horticultural or agri-silvicultural systems.” On-farm conservation involves conserving landraces within traditional farming systems and has been practised by farmers for millennia: Each season the farmers keep a proportion of harvested seed for re-sowing in the following year. Thus, the landrace is highly adapted to the local environment and is likely to contain locally adapted alleles or gene complexes.
Home garden conservation: Crops are grown (often as small populations) and the produce is used primarily for home consumption. Home garden conservation is a variation on on-farm conservation and may be defined as: “the sustainable management of genetic diversity of locally developed traditional crop varieties by individuals in their back-yard”. Orchard gardens, which are often expanded versions of kitchen gardens, can be valuable reserves of genetic diversity:
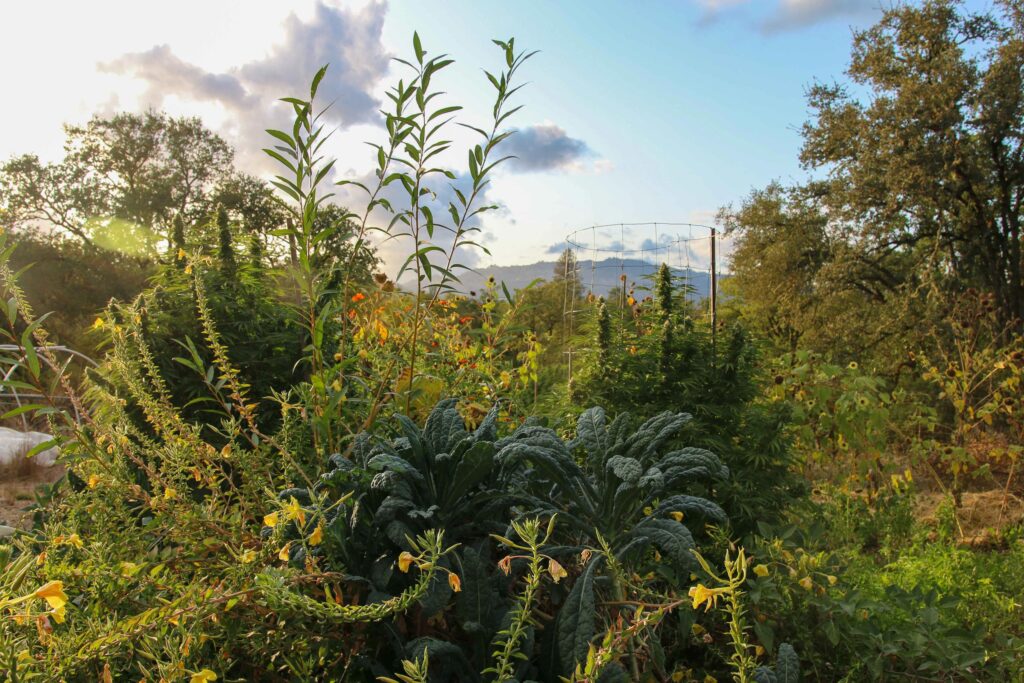
Home gardens and orchards are living gene banks where indigenous germplasm thrive. Home gardens are also important centers of experimentation, plant introduction, and crop improvement as well as refuges for unique genetic diversity. As the process of plant domestication and crop evolution is ongoing it can be expected that continuously new germplasm will develop: home gardens are a ‘window’ for introduction of, and experimentation with, genetic diversity.
Home gardens are dynamic systems; their structure, composition, and species and cultivar diversity are influenced by changes in the socioeconomic circumstances and cultural values of the households that maintain these gardens. Consequently, home gardens harbour significant amounts of unique and sometimes rare genetic diversity (at both the species and within-species/varietal level) that has evolved or been developed locally and that is of interest not only to the developers/farmers but also to the local and international conservation communities:
Home gardens can often maintain many more local varieties of some crops than might be found in larger scale production systems (common food crop examples include Sunflowers and Capsicum/ Peppers) or can maintain specific types (different varieties of sunflowers are grown/used for sprouting/edible micro-greens vs. hulling/shelled vs. in-shell sunflower seeds vs. bidrseed and/or ornamental varieties) that are not grown on a larger scale.

For example, the types of Capsicum maintained in home gardens may be quite different from those grown for commercial production and provide unique flavour, quality, season or other characteristics. Or the distinctive Asian home garden banana cultivars used for special purposes such as one type of banana that’s pickled & dried for medicinal use, with another type of green banana only used ceremonially (for offerings at the local Tet shrine). Since these kinds of local/traditional-use varieties are unlikely to be maintained on any scale in ex situ collections, home gardens may be the only reasonable way of maintaining the traits and diversity found.
More examples of the increased diversity found in home gardens: Andean potato farmers grow an average of 35 landrace potato varieties each, with an entire village/community growing as many as 50-70 types. Some Southeast Asian communities grow 78 varieties of rice in a single district. Chiapas farmers cultivate five races and a dozen local varieties of maize. Jivaro farmers, in one Amazon community, grow up to 100 varieties of manioc. A home garden in central Germany counts 105 species and varieties of plants in an area of about 750 square meters. A single Amazonian community near Iquitos, Peru cultivates 168 different species in 21 gardens…
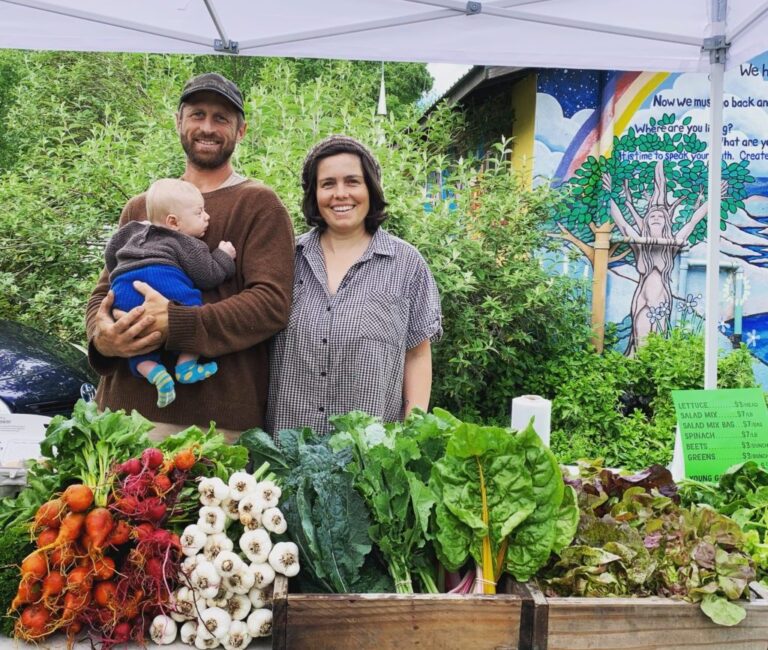
At the ecosystem level, the home garden provides a complex microenvironment that links more complex natural ecosystems with agricultural systems. It has been noted that home gardens tend to mimic the natural structure of forest systems, with the crucial difference that nearly all the species found in a home garden are used.
Home gardens tend to be complex, multi-storeyed environments with very high species diversity and a wide range of very varied ecological micro-niches: For example, the structure of the typical Cuban home garden varies depending upon the topography, but always maintains all 4 strata of vegetation that appear in an intact mature ecosystem (subterranean/ground-layer, herbaceous, bushes and trees), with cultivated species, weeds and wild species being characteristic of each strata, although the cultivated species constitute the greatest proportion.

Home gardens provide additional ecosystem services which benefit the larger agricultural systems and the health and well being of the household:
Home gardens often provide protected and enriched environments for varieties that may have been more susceptible to biotic and abiotic stresses when grown in open fields. Other ecosystem services provided by home gardens include soil enrichment, improved water retention, and a habitat for pollinators.
Home gardens are a good example of how humans can positively cause ecological niche differentiation which increases the total productivity of the agroecosystem.

Home gardens also act as important refuges for the survival, conservation and biodiversity maintenance of many endangered species that are in the process of losing their natural habitat:
For example in the Alta Verapaz region of Cuba, where there is increasingly limited access to the native forest due to deforestation and land tenure change, farmers often use their home garden to grow plants they used to gather from the wild. Some useful wild species are still gathered in their native habitat; however it is common to find important species growing in home gardens particularly if the species are not available in the market. Species inventories done in home gardens of the region indicated they are repositories of species growing in the natural forest.
Home gardens can be important for in situ conservation of wild species whose habitat is threatened, as well as for species in the process of domestication.
Regions Of Provenance aka Local EcoRegions
The growing realization that a species of plant can develop unique characteristics based on the ecological characteristics unique to a particular ecology/ecosystem has been acknowledged and codified by the Region Of Provenance model (formalized in 2013 European Union plant protection and reforestation regulations):
The Region of Provenance (RoP) for a species is an area (or group of areas) where the ecological features are homogeneous/similar,
The principle is that when planning/sourcing any new agricultural/crop endeavor/ project, it’s best to use reproductive materials/genetics from the Region(s) Of Provenance with the highest possibility of adaptation and climatic correspondence to the new target location/ cultivation area:
Always aim to select genetics/source material from those Regions Of Provenance (aka local ecoregions) that have similar climatic and environmental characteristics (for example: similar latitude/photoperiod, elevation/altitude, and soil type) as the new area being cultivated.
How you can help:
No-one can do everything, everyone can do something…
You too can do a great deal for diversity!:
Agricultural diversity will not be saved unless it is used. It is only through use that diversity is appreciated. In order to conserve landraces in their ‘centres of origin’ priority must be given to understanding and increasing their utilization: Markets for traditional landraces, products made from traditional landraces and markets for traditional heirloom landrace seed need to be expanded and consumer awareness & appreciation raised about the many benefits of biodiversity…
Additional cultural benefits of homegrown biodiversity:
In Peru, certain native local heirloom landrace potatoes command a higher price because they taste better and are more prestigious as gifts…
Home garden owners with higher levels of education tend to cultivate a greater number of species in their gardens… (i.e. the research suggests that farmers are capable of perceiving greater benefit in managing a greater number of species…)
“Traditional seed cultivation and production” was declared an intangible cultural heritage by UNESCO in 2014…
Home gardens are often the focal point of a household’s social interactions within the family and with visitors. One of the important functions that home gardens perform is to keep knowledge of varieties and uses of diversity alive from generation to generation. In home gardens children and visitors can learn from the family experts in different types of diversity and its uses. These can be nutritional, commercial, aesthetic, and spiritual. Home gardens around the world serve as important genetic refuges for the ‘heirloom crop varieties’ that were valued and maintained in the family but had little place in commercial markets. Households were also able to exchange their home garden varieties as part of the social visits. Sharing and exchanging plant genetic resources are common features of visits between households.
Some ways you can help contribute to the global movement to preserve, protect and regenerate our planet’s cannabis hemp genetic diversity & planetary gene pool:
By collecting, growing, purchasing, or passing on landrace seeds, knowledge, and enthusiasm…
Check out our current selection of landrace seeds from around the globe!
- Support our Landrace Biodiversity Regeneration Project!
When selecting/shopping for seeds, try to select strains/varieties whose genetic provenance comes from an eco-region/provenance that has similar characteristics to the local eco-region where your grow site is located:
As a general rule, genetics should come from a region that has similar climate, rainfall, elevation/altitude and soil-type characteristics as your local site, i.e. genetics should not come from regions with a wetter, drier, or warmer climate than the one at your local grow site.
You can play a valuable role in helping conserve on-farm diversity by turning (part of) your garden into a genetics preservation & regeneration living-genebank reserve: plant and propagate as many varieties/types as possible! (The security of genetic resource conservation is strengthened if farmers are actually using and helping regenerate plant varieties…).
When planning/planting for diversity, it’s important to include diversity in the number of varieties, as well as large a population size of each variety as possible (diversity is increased as the population size increases and when the source seed comes from as many different individual plants/genotypes as possible).
Breeders: you can help by expanding the focus of your breeding efforts to include ecology, weed science, soil erosion potential, and nutrient use efficiency.
Join a seed/strain regeneration & preservation project! (You can Contact Us to help get connected with an upcoming regeneration project…)
~ ~ ~

Citations/info-sources
Cannabis Domestication, Breeding History, Present-day Genetic Diversity, and Future Prospects, Robert C. Clarke & Mark D. Merlin, Critical Reviews in Plant Sciences, 35:5-6, 293-327 (2016), http://dx.doi.org/10.1080/07352689.2016.1267498, Oxfordshire England
Latitudinal Adaptation and Genetic Insights Into the Origins of Cannabis sativa L., Zhang Q, Chen X, Guo H, Trindade LM, Salentijn EMJ, Guo R, Guo M, Xu Y and Yang M, Frontiers in Plant Science 9:1876, 2018 doi: 10.3389/fpls.2018.01876, Lausanne Switzerland
A classification of endangered high-THC cannabis (Cannabis sativa subsp. indica) domesticates and their wild relatives, McPartland JM & Small E. PhytoKeys 177: 81–112. https://doi.org/10.3897/phytokeys.144.46700, 2020, Bulgaria
Talking Landrace Strains with Franco Loja, Franco Loja w/ High Times magazine, January 25, 2017
Cannabis Botany & Evolution, video interview with Robert Clarke & Project CBD, 2016
Landraces: A review of definitions and classifications, AC Zeven, Euphytica 104: 127–139, 1998, the Netherlands
Toward an Evolved Concept of Landrace, Casañas F, Simó J, Casals J and Prohens J., Frontiers in Plant Science 8:145 (2017), doi: 10.3389/fpls.2017.00145, Lausanne Switzerland
New Insights Into Ancient Grain, Richard Blaustein, BioScience 63: 421–426, 2013, doi:10.1525/bio.2013.63.6.4, Washington DC
Resource Book for the Preparation of National Plans for Conservation of Crop Wild Relatives and Landraces, Maxted N, Magos Brehm J and Kell S, FAO (UN Food & Agriculture Organization), 2013, Rome
Prohibition & Resistance: A Socio-Political Exploration of the Changing Dynamics of the Southern African Cannabis Trade, c. 1850–The Present, Craig Paterson, Rhodes University Masters Thesis, 2009, Grahamstown South Africa
Regions of provenance for reproductive materials of the three main forest species of Abruzzi, Marchi, Castaldi, Di Silvestro, Contu & Ducci, Journal of Maps, 12:sup1, 94-97, 2016, DOI: 10.1080/17445647.2016.1159886, Oxfordshire England
Regulation on the production and making available on the market of plant reproductive material (plant reproductive material law), EU European Union 2013
European landraces on- farm conservation, management and use, Veteläinen M, Negri V and Maxted N., Bioversity Technical Bulletin No. 15, 2009, Bioversity International aka International Plant Genetic Resources Institute (IPGRI), Rome, Italy.
Importance of Landraces in Cereal Breeding for Stress Tolerance, Marone,D.;Russo,M.A.; Mores, A.; Ficco, D.B.M.; Laidò, G.; Mastrangelo, A.M.; Borrelli, G.M., Plants 2021,10,1267. https://doi.org/ 10.3390/plants10071267, Basel, Switzerland
Ecotypes, Northern Arizona University, accessed from https://www2.nau.edu/~gaud/bio300w/ecotype.htm on 5/16/2022, Flagstaff Arizona
Cannabis: A Complete Guide, Ernest Small, CRC Press; 1st edition (November 17, 2016)
Ecological Restoration: Principles, Values and Structure of an Emerging Profession, Clewell & Aronson, Society for Ecological Restoration International & Island Press, 2007, Washington DC
Strategies for the Conservation of Plant Genetic Resources for Food and Agriculture, Paula Westmoreland, Institute for Agriculture & Trade Policy, April 1999, accessed from https://www.iatp.org/sites/default/files/Strategies_for_the_Conservation_of_Plant_Genet.htm on 1/3/2023, Minneapolis Minnesota
Home Gardens and In Situ Conservation of Plant Genetic Resources in Farming Systems, edited by JW Watson & PB Eyzaguirre, Proceedings of the Second International Home Gardens Workshop, International Plant Genetic Resources Institute, July 17-19 2001, Maccarese Italy
Cite this page as: Landraces, Local Ecotypes & EcoRegions Of Provenance Primer: A Business Case For Biodiversity, Motor City Seeds Team, January 15 2023, accessed from https://motorcityseeds.org/landraces-ecotypes-primer/, Detroit Michigan